Please see Reproduction in Organisms Class 12 Biology Revision Notes provided below. These revision notes have been prepared as per the latest syllabus and books for Class 12 Biology issues by CBSE, NCERT, and KVS. Students should revise these notes for Chapter 1 Reproduction in Organisms daily and also prior to examinations for understanding all topics and to get better marks in exams. We have provided Class 12 Biology Notes for all chapters on our website.
Chapter 1 Reproduction in Organisms Class 12 Biology Revision Notes
IMPORTANT TERMS
• Genetics: Study of inheritance, heredity and variation of characters or Study of genes and chromosomes.
• Inheritance: Transmission of characters from parents to progeny. It is the basis of Heredity.
• Variation: Difference between parents and offspring.
• Character: A heritable feature among the parents & offspring. E.g. Eye colour.
• Trait: Variants of a character. E.g. Brown eye, Blue eye.
• Allele: Alternative forms of a gene. E.g. T (tall) and t (dwarf) are two alleles of a gene for the character height.
• Homozygous: The condition in which chromosome pair carries similar alleles of a gene. Also known as pure line (True breeding). E.g. TT, tt, YY, yy etc.
• Heterozygous: The condition in which chromosome pair carries dissimilar alleles of a gene. E.g. Tt, Yy etc.
• Dominant character: The character which is expressed in heterozygous condition. It indicates with capital letter.
• Recessive character: The character which is suppressed in heterozygous condition. It indicates with small letter.
• Phenotype: Physical expression of a character.
• Genotype: Genetic constitution of a character.
• Hybrid: An individual produced by the mating of genetically unlike parents.
• Punnett square: A graphical representation to calculate probability of all genotypes of offspring in a genetic cross.
MENDEL’S LAWS OF INHERITANCE
Gregor Mendel is the Father of genetics.
He conducted some hybridization experiments on garden peas (Pisum sativum) for 7 years (1856-1863).
Steps in making a cross (Deliberate mating) in pea:
• Selection of 2 pea plants with contrasting characters.
• Emasculation: Removal of anthers of one plant to avoid self-pollination. This is female parent.
• Pollination: Collection of pollen grains from the male parent and transferring to female parent.
• Collection & germination of seeds to produce offspring.
Mendel selected 7 pairs of true breeding pea varieties:

INHERITANCE OF ONE GENE
Monohybrid cross: A cross involving 2 plants differing in one character pair. E.g. Mendel crossed tall and dwarf pea plants to study the inheritance of one gene.
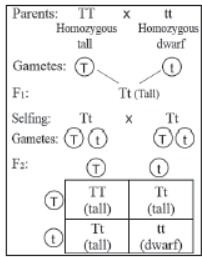
Monohybrid phenotypic ratio:
3 Tall: 1 Dwarf = 3:1
Monohybrid genotypic ratio:
1 Homozygous tall (TT)
2 Heterozygous tall (Tt)
1 Homozygous dwarf (tt)
= 1:2:1
Mendel made similar
observations for other pairs of
traits. He proposed that some
factors were inherited from
parent to offspring. Now it is called as genes.
Do not use T for tall and d for dwarf because it is difficult
to remember whether T & d are alleles of same gene or not.
The F1 (Tt) when self-pollinated, produces gametes T and t in equal proportion. During fertilization, pollen grains of T have 50% chance to pollinate eggs of T & t. Also, pollen grains of t have 50% chance to pollinate eggs of T and t.
1/4th of the random fertilization leads to TT (¼ TT).
1/2 (2/4) of the random fertilization leads to Tt (½ Tt).
1/4th of the random fertilization leads to tt (¼ tt).
random fertilization leads to tt (¼ tt).
Tt x Tt
Binomial expression = (ax + by) 2
Hence (½ T + ½ t) 2 = (½ T + ½ t) (½ T + ½ t)
= ¼ TT + ¼ Tt + ¼ Tt + ¼ tt
= ¼ TT + ½ Tt + ¼ tt
Mendel self-pollinated the F2 plants. He found that dwarf F2 plants continued to generate dwarf plants in F3 & F4. He concluded that genotype of the dwarfs was homozygous- tt.
Backcross and Testcross
• Backcross: Cross between a hybrid and its any parent.
• Testcross: Crossing of an organism with dominant
phenotype to a recessive individual. E.g.

Backcross and Testcross
• Backcross: Cross between a hybrid and its any parent.
• Testcross: Crossing of an organism with dominant
phenotype to a recessive individual. E.g.
Hence
monohybrid
test cross ratio=
Test cross is used to find out the unknown genotype of a character. E.g..

Mendel conducted test cross to determine the F2 genotype.
Mendel’s Principles or Laws of Inheritance
1. First Law (Law of Dominance)
• Characters are controlled by discrete units called factors.
• Factors occur in pairs.
• In a dissimilar pair of factors, one member of the pair dominates (dominant) the other (recessive).
2. Second Law (Law of Segregation)
“During gamete formation, the factors (alleles) of a character pair present in parents segregate from each other such that a gamete receives only one of the 2 factors”.
Homozygous parent produces similar gametes.
Heterozygous parent produces two kinds of gametes.
INHERITANCE OF TWO GENES
Dihybrid cross: It is a cross between two parents differing in 2 pairs of contrasting characters. E.g. Cross b/w pea plant with homozygous round shaped & yellow coloured seeds (RRYY) and wrinkled shaped & green coloured seeds (rryy). .

On observing the F2, Mendel found that yellow and green colour segregated in a 3:1 ratio.
Round & wrinkled seed shape also segregated in a 3:1 ratio.
Dihybrid Phenotypic ratio= 9 Round yellow: 3 Round
green: 3 Wrinkled yellow: 1 Wrinkled green = 9:3:3:1
The ratio of 9:3:3:1 can be derived as a combination series of
3 yellow: 1 green, with 3 round: 1 wrinkled.
i.e. (3: 1) (3: 1) = 9: 3: 3: 1
Dihybrid genotypic ratio: 1:2:1:2:4:2:1:2:1
RRYY =1 RRYy =2 RrYY =2
RrYy =4 RRyy =1 Rryy =2
rrYY =1 rrYy =2 rryy =1
Mendel’s 3rd Law: Law of Independent Assortment
– It is based on the results of dihybrid crosses.
– It states that “When two pairs of traits are combined in a hybrid, segregation of one pair of characters is independent of the other pair of characters”.
The concept of dominance
– Every gene contains information to express a particular trait.
– In heterozygotes, there are 2 types of alleles:
o Unmodified (normal or functioning) allele: It is generally dominant and represents original phenotype.
o Modified allele: It is generally recessive.
– E.g. Consider a gene that contains information for producing an enzyme. Normal allele of that gene produces a normal enzyme. Modified allele is responsible for production of
(i) Normal/less efficient enzyme or
(ii) A non-functional enzyme or
(iii) No enzyme at all
In the first case: The modified allele will produce the same phenotype like unmodified allele. Thus, modified allele is equivalent to unmodified allele.
In 2nd and 3rd cases: The phenotype will dependent only on the functioning of the unmodified allele. Thus the modified allele becomes recessive.
1. Incomplete Dominance
– It is an inheritance in which heterozygous offspring shows intermediate character b/w two parental characteristics.
– E.g. Flower colour in snapdragon (dog flower or Antirrhinum sp.) and Mirabilis jalapa (4’O clock plant).

Here, cross between
homozygous red &
white produces pink
flowered plant. Thus
phenotypic & genotypic
ratios are same.
Phenotypic ratio=
1 Red: 2 Pink: 1 White
Genotypic ratio=
1 (RR): 2 (Rr): 1(rr)
This means that R was not completely dominant over r.
– Pea plants also show incomplete dominance in other traits.
2. Co-dominance
– It is the inheritance in which both alleles of a gene are expressed in a hybrid. E.g. ABO blood grouping in human.
– ABO blood groups are controlled by the gene I.
– This gene controls the production of sugar polymers (antigens) that protrude from plasma membrane of RBC.
– The gene I has three alleles IA, IB & i.
– IA and IB produce a slightly different form of the sugar while allele i doesn’t produce any sugar.

When IA and IB are present together, they both express their own types of sugars. This is due to co-dominance.
3. Multiple allelism
– It is the presence of more than two alleles of a gene to govern same character.
– E.g. ABO blood grouping (3 alleles: IA, IB & i).
– In an individual, only two alleles are present. Multiple alleles can be found only in a population.
4. Polygenic inheritance
– It is the inheritance in which some traits are controlled by several genes (multiple genes).
– E.g. human skin colour, human height etc.
– It considers the influence of environment.
– In a polygenic trait, the phenotype reflects the contribution of each allele, i.e., the effect of each allele is additive.
Human skin colour :
– Assume that 3 genes A, B, C control human skin colour.
The dominant forms A, B & C responsible for dark skin colour and recessive forms a, b & c for light skin colour.
– Genotype with all the dominant alleles (AABBCC) gives
darkest skin colour.
Genotype with all the recessive alleles (aabbcc) gives
lightest skin colour.
Therefore, genotype with 3 dominant alleles and 3 recessive alleles gives an intermediate skin colour.
– Thus, number of each type of alleles determines the darkness or lightness of the skin.
5. Pleiotropy
– Here, a single gene exhibits multiple phenotypic expressions. Such a gene is called pleiotropic gene.
– In most cases, the mechanism of pleiotropy is the effect of a gene on metabolic pathways which contributes towards different phenotypes.
– E.g. Starch synthesis in pea, sickle cell anaemia, phenylketonuria etc.
– In Phenylketonuria & sickle cell anaemia, the mutant gene has many phenotypic effects. E.g. Phenylketonuria causes mental retardation, reduction in hair and skin pigmentation.
Starch synthesis in pea plant:
– Starch is synthesized effectively by BB gene. Therefore, large starch grains are produced. bb have lesser efficiency in starch synthesis and produce smaller starch grains.
– Starch grain size also shows incomplete dominance.
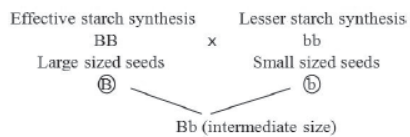
CHROMOSOMAL THEORY OF INHERITANCE
Mendel’s work remained unrecognized till 1900 because,
• Communication was not easy.
• His mathematical approach was new and unacceptable.
• The concept of genes (factors) as stable and discrete units could not explain the continuous variation seen in nature.
• He could not give physical proof for the existence of factors.
In 1900, de Vries, Correns & von Tschermak independently rediscovered Mendel’s results.
Chromosomal Theory of Inheritance (1902):
– Proposed by Walter Sutton & Theodore Boveri.
– They said that pairing & separation of a pair of chromosomes lead to segregation of a pair of factors they carried.
– Sutton united chromosomal segregation with Mendelian principles and called it the chromosomal theory of inheritance. It states that,
• Chromosomes are vehicles of heredity.
• Two identical chromosomes form a homologous pair.
• Homologous pair segregates during gamete formation.
• Independent pairs segregate independently of each other.
Genes (factors) are present on chromosomes. Hence genes and chromosomes show similar behaviours.

Thomas Hunt Morgan proved chromosomal theory of inheritance using fruit flies (Drosophila melanogaster).
It is the suitable material for genetic study because,
• They can grow on simple synthetic medium.
• Short generation time (life cycle: 12-14 days).
• Breeding can be done throughout the year.
• Hundreds of progenies per mating.
• Male and female flies are easily distinguishable. E.g. Male is smaller than female.
• It has many types of hereditary variations that can be seen with low power microscopes.
LINKAGE AND RECOMBINATION
Linkage is the physical association of two or more genes on a chromosome. They do not show independent assortment.
Recombination is the generation of non-parental gene combinations. It occurs due to independent assortment or crossing over.
Morgan carried out several dihybrid crosses in Drosophila to study sex-linked genes. E.g.
Cross 1: Yellow-bodied, white-eyed females X
Brown-bodied, red-eyed males (wild type)
Cross 2: White-eyed, miniature winged X
Red eyed, large winged (wild type)
Morgan intercrossed their F1 progeny. He found that
• The two genes did not segregate independently and the F2 ratio deviated from the 9:3:3:1 ratio.
• Genes were located on the X chromosome.
• When two genes were situated on the same chromosome,the proportion of parental gene combinations was much higher than the non-parental type. This is due to linkage.
• Genes of white eye & yellow body were very tightly linked and showed only 1.3% recombination.
• Genes of white eye & miniature wing were loosely linked and showed 37.2% recombination.
• Tightly linked genes show low recombination. Loosely linked genes show high recombination.
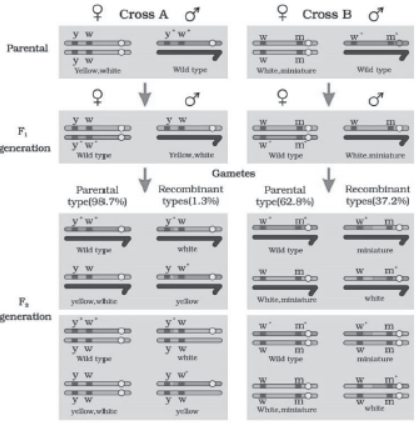
Alfred Sturtevant used the recombination frequency between gene pairs for measuring the distance between genes and ‘mapped’ their position on the chromosome.
Genetic maps are used as a starting point in the sequencing of genomes. E.g. Human Genome Project.
SEX DETERMINATION
– The chromosomes that are involved in sex determination are called sex chromosomes (allosomes). They include X & Y chromosomes.
– Autosomes are chromosomes other than sex chromosomes.
Number of autosomes is same in males and females.
– Henking (1891) studied spermatogenesis in some insects and observed that 50 % of sperm received a nuclear structure after spermatogenesis, and other 50 % sperm did
not receive it. Henking called this structure as the X body (now it is called as X-chromosome).
Mechanism of sex determination
a. XX-XO mechanism: Here, male is heterogametic, i.e.
XO (Gametes with X and gametes without X) and female is homogametic, i.e. XX (all gametes are with Xchromosomes).
E.g. Many insects such as grasshopper.
b. XX-XY mechanism: Male is heterogametic (X & Y) and female is homogametic (X only). E.g. Human &Drosophila.
c. ZZ-ZW mechanism: Male is homogametic (ZZ) and female is heterogametic (Z & W). E.g. Birds.
XX-XO & XX-XY mechanisms show male heterogamety.
ZZ-ZW mechanism shows female heterogamety.
Sex Determination in Humans (XX-XY type)
• Human has 23 pairs of chromosomes (22 pairs of autosomes and 1 pair of sex chromosomes).
• A pair of X-chromosomes (XX) is present in the female, whereas X and Y chromosomes are present in male.
• During spermatogenesis, males produce 2 types of gametes: 50 % with X-chromosome and 50 % with Y-chromosome.
• Females produce only ovum with an X-chromosome.
• There is an equal probability of fertilization of the ovum with the sperm carrying either X or Y chromosome.
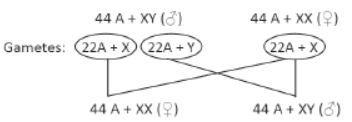
The sperm determines whether the offspring male or female.
Sex determination in honeybee
– It is based on the number of sets of chromosomes an individual receives.
– Fertilised egg develops as a female (queen or worker).
– An unfertilised egg develops as a male (drone). It is called parthenogenesis.
– Therefore, the females are diploid (32 chromosomes) and males are haploid (16 chromosomes). This is called as haplodiploid sex determination system.
– In this system, the males produce sperms by mitosis. They do not have father and thus cannot have sons, but have a grandfather and can have grandsons.
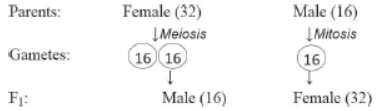
MUTATION, PEDIGREE ANALYSIS AND GENETIC DISORDERS
MUTATION
It is a sudden heritable change in DNA sequences resulting in changes in the genotype and the phenotype of an organism.
Mutation is 2 types:
• Point mutation: The mutation due to change (substitution) in a single base pair of DNA. E.g. sickle cell anaemia.
• Frame-shift mutation: It is the deletion or insertion of base pairs resulting in the shifting of DNA sequences.
• Loss (deletion) or gain (insertion/ duplication) of DNA segment cause Chromosomal abnormalities (aberrations).
• Chromosomal aberrations are seen in cancer cells.
• The agents which induce mutation are called mutagens.
They include
– Physical mutagens: UV radiation, α, β, γ rays, X-ray etc.
– Chemical mutagens: Mustard gas, phenol, formalin etc.
PEDIGREE ANALYSIS
– In human, control crosses are not possible. So the study of family history about inheritance is used.
– Such an analysis of genetic traits in several generations of a family is called pedigree analysis.
– The representation or chart showing family history is called family tree (pedigree).
– In human genetics, pedigree study is utilized to trace the inheritance of a specific trait, abnormality or disease.
Symbols used in pedigree analysis

GENETIC DISORDERS
The disorders due to change in genes or chromosomes.
2 types: Mendelian disorders & Chromosomal disorders.
1. Mendelian Disorders
• It is caused by alteration or mutation in the single gene.
• E.g. Haemophilia, Colour blindness, Sickle-cell anaemia, Phenylketonuria, Thalassemia, Cystic fibrosis etc.
• The pattern of inheritance of Mendelian disorders can be traced in a family by the pedigree analysis.
• Mendelian disorders may be dominant or recessive.
• Pedigree analysis helps to understand whether the trait is dominant or recessive.

Pedigree analysis of
(A) Autosomal dominant trait (E.g. Myotonic dystrophy)
(B) Autosomal recessive trait (E.g. Sickle-cell anaemia)
Haemophilia (Royal disease):
• It is a sex linked (X-linked) recessive disease.
• In this, a protein involved in the blood clotting is affected.
• A simple cut results in non-stop bleeding.
• The disease is controlled by 2 alleles, H & h. H is normal allele and h is responsible for haemophilia.
XHXH | Normal female |
XHXh | Heterozygous female (carrier). She may transmit the disease to sons. |
XhXh | Hemophilic female |
XHY | Normal male |
XhY | Hemophilic male |
• In females, haemophilia is very rare because it happens only when mother is at least carrier and father haemophilic (unviable in the later stage of life).
• Queen Victoria was a carrier of hemophilia. So her family pedigree shows many haemophilic descendants.
Colour blindness:
• It is a sex-linked (X-linked) recessive disorder due to defect in either red or green cone of eye. It results in failure to discriminate between red and green colour.
• It is due to mutation in some genes in X chromosome.
• It occurs in 8% of males and only about 0.4% of females.
This is because the genes are X-linked.
• Normal allele is dominant (C). Recessive allele (c) causes colour blindness.
• The son of a heterozygous woman (carrier, XCXc) has a 50% chance of being colour blind.
• A daughter will be colour blind only when her mother is at least a carrier and her father is colour blind (XcY).
Sickle-cell anaemia :
• This is an autosome linked recessive disease.
• It can be transmitted from parents to the offspring when both the partners are carrier (heterozygous) for the gene.
• The disease is controlled by a pair of allele, HbA and HbS.
Homozygous dominant (HbAHbA): normal Heterozygous (HbAHbS): carrier; sickle cell trait Homozygous recessive (HbSHbS): affected
• The defect is caused by the substitution of Glutamic acid (Glu) by Valine (Val) at the sixth position of the β-globin chain of the haemoglobin (Hb). (Image 5)
• This is due to the single base substitution at the sixth codon of the β-globin gene from GAG to GUG.
• The mutant Hb molecule undergoes polymerization under low oxygen tension causing the change in shape of the RBC from biconcave disc to elongated sickle like structure.
Phenylketonuria:
• An inborn error of metabolism.
• Autosomal recessive disease.
• It is due to mutation of a gene that codes for the enzyme phenyl alanine hydroxylase. This enzyme converts an amino acid phenylalanine into tyrosine.
• The affected individual lacks this enzyme. As a result, phenylalanine accumulates and converts into phenyl pyruvic acid and other derivatives.
• They accumulate in brain resulting in mental retardation.
These are also excreted through urine because of poor absorption by kidney.
Thalassemia:
• An autosome-linked recessive blood disease.
• It is transmitted from unaffected carrier (heterozygous) parents to offspring.
• It is due to mutation or deletion.
• It results in reduced synthesis of a or b globin chains of haemoglobin. It forms abnormal haemoglobin and causes anaemia.
• Based on the chain affected, thalassemia is 2 types: o a Thalassemia: Here, production of a globin chain is affected. It is controlled by two closely linked genes HBA1 & HBA2 on chromosome 16 of each parent.
Mutation or deletion of one or more of the four genes causes the disease. The more genes affected, the less a globin molecules produced.
o b Thalassemia: Here, production of b globin chain is affected. It is controlled by a single gene HBB on chromosome 11 of each parent. Mutation of one or both the genes causes the disease.
• Thalassemia is a quantitative problem (synthesise very less globin molecules). Sickle-cell anaemia is a qualitative problem (synthesise incorrectly functioning globin).
2. Chromosomal disorders They are caused due to absence or excess or abnormal arrangement of one or more chromosomes. 2 types:
a. Aneuploidy: The gain or loss of chromosomes due to failure of segregation of chromatids during cell division.
b. Polyploidy (Euploidy): It is an increase in a whole set of chromosomes due to failure of cytokinesis after telophase stage of cell division. This is very rare in human but often seen in plants.
Examples for chromosomal disorders
• Down’s syndrome: It is the presence of an additional copy of chromosome number 21 (trisomy of 21).
Genetic constitution: 45 A + XX or 45 A + XY (i.e. 47 chromosomes).
Features:
o They are short statured with small round head.
o Broad flat face.
o Furrowed big tongue and partially open mouth.
o Many “loops” on finger tips.
o Broad palm with characteristic palm simian crease.
o Retarded physical, psychomotor & mental development.
o Congenital heart disease.
• Klinefelter’s Syndrome: It is the presence of an additional copy of X-chromosome in male (trisomy).
Genetic constitution: 44 A + XXY (i.e. 47 chromosomes).
Features:
o Overall masculine development. However, the feminine development is also expressed. E.g.
Development of breast (Gynaecomastia).
o Sterile.
o Mentally retarded.
• Turner’s syndrome: This is the absence of one X chromosome in female (monosomy).
Genetic constitution: 44 A + X0 (i.e. 45 chromosomes).
Features:
o Sterile, Ovaries are rudimentary.
o Lack of other secondary sexual characters.
o Dwarf.
o Mentally retarded.